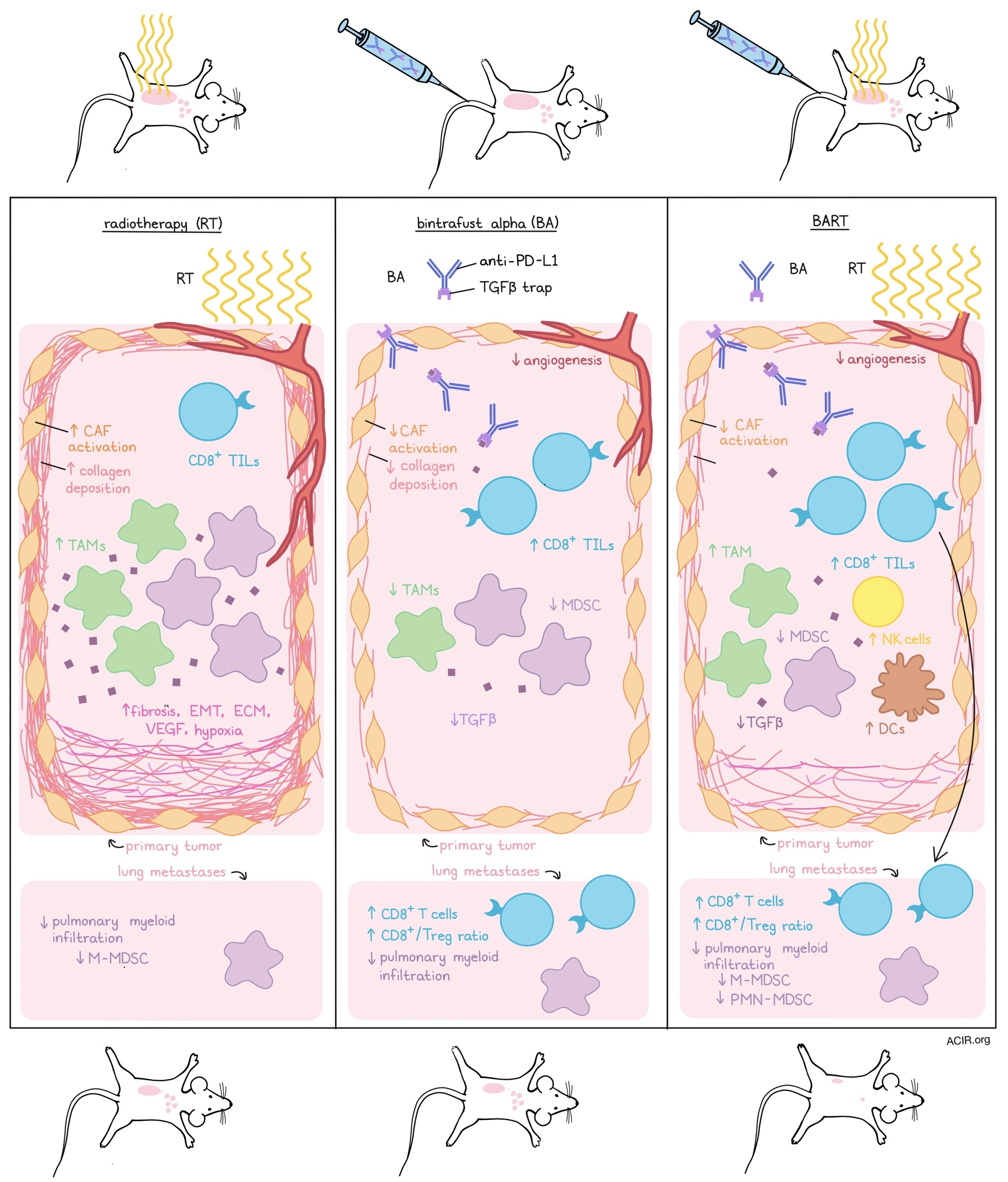
Since radiotherapy (RT) can boost immune priming, it can be a great tool to convert immune “cold” tumors into “hot” immune environments, making way for effective checkpoint inhibition therapies. However, RT may also remodel the tumor microenvironment (TME), creating barriers to immune infiltration and antitumor effects. Given that one of the main contributors to TME remodeling is the TGFβ pathway, combining RT with neutralization of TGFβ and checkpoint blockade may effectively treat cold tumors. Lan, Moustafa, Knoll, and Xu, et al. investigated whether the addition of bintrafusp alfa (BA), a bifunctional fusion protein combining the extracellular domain of the TGFβRII (functioning as a TGFβ “trap”) and an anti-PD-L1 antibody, to RT (BART) can increase therapy efficacy. Their findings were recently published in Cancer Cell.
The researchers evaluated their therapeutic strategy in multiple mouse models (KPC, LLC, GL261, 4T1) that lack TIL and present with features of fibrosis, inflamed TME, and generally have limited responses to checkpoint blockade and/or radiation. In these models, BA and RT monotherapy reduced tumor burden, reduced tumor growth, and increased tumor regression. BART significantly increased these effects, resulting in prolonged survival in some of the models.
To determine what factors in the TME were affected by BART, resulting in the enhanced antitumor effects, the researchers studied fibrosis in the TME in the EMT-6 model. BA reduced α-smooth muscle actin and collagen deposition, indicative of reduced cancer-associated fibroblast (CAF) activation, and increased CD8+ TIL infiltration and repertoire. RT, on the other hand, increased activation of CAFs and deposition of collagen, which could be reversed by BART. Angiogenesis in the TME was reduced by BA, an effect that was enhanced when combined with RT.
Looking at the immune infiltrate in treated tumors, BART increased the intratumoral density of CD8+ T cells, dendritic cells (DCs), and activated NK cells, while it decreased the density of myeloid-derived suppressor cells (MDSCs). Showing the essential role of the immune infiltrate on the therapeutic effects of BART, antibody depletion of CD8+ T cells or NK cells resulted in a reduction of the inhibition of tumor growth and reduced survival.
The researchers then investigated gene expression changes induced by the therapy in the 4T1 model using RNAseq and curated gene expression signatures. Both BA and BART decreased TGFβ signature scores, and BA also decreased predictive scores of RT-induced fibrosis, extracellular matrix, epithelial-to-mesenchymal transition (EMT), vascular endothelial growth factor (VEGF) pathways, and hypoxia signatures. On the other hand, RT alone increased EMT and hypoxia signatures, while BART reversed these effects. Immune signatures also changed, with BA increasing the stimulator of interferon genes (STING) and slightly increasing IFN-associated signatures, while RT and BART upregulated these signatures even more, with RT resulting in the highest expression. Tumor-associated macrophage signatures were increased after RT, decreased after BA, and partially increased after BART. Additionally, BART increased NK cell signatures, and both BA and BART decreased MDSC signature scores, with the most prominent effect caused by BART.
The researchers then took the signatures of BART-regulated genes in the mouse model to analyze its prognostic significance for patients. Patient data from a Phase II trial using atezolizumab (anti-PD-L1) in patients with metastatic urothelial cancer were clustered based on the discovered BART-regulated genes. This resulted in three patient groups, which showed variable survival outcomes, in contrast to clustering based on immune signatures or monotherapy-related genes. Patients in cluster 3 had improved outcomes, reduced expression of TGFβ signaling-related genes, and increased expression of immune activation genes, suggesting prognostic significance of this gene signature.
Studying the immune effects of BART further, the 4T1 model was used to assess whether the treatment could enhance antitumor responses and affect metastases. Tumor-bearing mice with lung metastases were treated systemically with BA, while the primary tumor received RT, using a small animal radiation research platform that mimics localized RT in patients. While the monotherapies reduced the tumor burden of the primary tumors, BART reduced both primary and lung tumor burden, more so than BA alone, suggesting a systemic effect.
Given that these systemic effects might be caused by the induction of antitumor T cell responses, the researchers assessed the immune infiltrates in metastatic lesions. The density of CD8+ T cells and the ratio of CD8+ T cells to Tregs increased after BA and BART, with the most significant increase after BART. When CD8+ T cells or NK cells were depleted, the inhibition of primary tumor growth induced by BART was partially abrogated. Additionally, depletion of CD8+ T cells abrogated the BART-induced tumor reduction effects on metastases, suggesting the essential role of CD8+ T cells in the abscopal effects of BART.
The 4T1 lung metastasis model is characterized by pulmonary myeloid cell infiltration, but this was reduced after BA and, more significantly, BART. BART decreased the total number of polymorphonuclear (PMN)-MDSCs, in contrast to monotherapies. Additionally, RT and BART reduced the number of monocytic (M)-MDSCs.
To investigate the effects of BA on RT-induced lung fibrosis, mice were treated with RT, resulting in increased lung density and reduced lung volume size, indicative of tissue remodeling. The addition of BA treatment resulted in a lower lung density and protected the lung volume, while anti-PD-L1 or a TGFβ trap alone did not affect it. In addition, post-RT BA reduced lung fibrosis, preserved lung tissue architecture, decreased collagen deposition, normalized alveoli, and protected pulmonary function. Using single-cell RNAseq, mouse lungs treated with RT or BART were subjected to tSNE plotting of cellular populations. Fibrosis-associated markers were then used to identify cell populations critical for the lung fibrosis seen after RT. PD-L1 was mainly expressed at high levels in lipofibroblast-like clusters and in some endothelial clusters, which also expressed TGFβ. These data suggest that BA may target the TGFβ trap to relevant populations that also express PD-L1.
Together, these data show that radiotherapy and co-targeting TGFβ and PD-L1 can stimulate antitumor immune responses and overcome immune barriers in immunologically cold tumors, creating an effective “in situ vaccine”, while simultaneously overcoming some of the adverse fibrotic effects caused by RT in the TME. These data support the clinical translation of this approach.
Write-up by Maartje Wouters, image by Lauren Hitchings
Meet the researcher
This week, senior author Amir Abdollahi answered our questions.
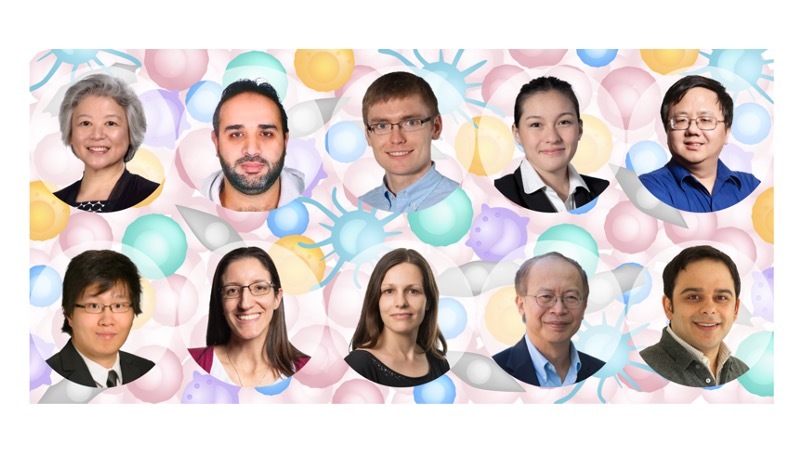
What prompted you to tackle this research question?
Despite the rapid progress of immune oncology (IO), unfortunately, still a large fraction of cancer patients do not benefit from immunotherapy. In certain entities such as primary brain tumors (glioblastoma) and pancreatic cancer, the prognosis remains poor, despite evaluation of several treatment escalation strategies. Our motivation was therefore to discover a potentially universal strategy to reconstitute tumor immune surveillance to cure these therapy-resistant tumors. Modern high-precision radiotherapy (RT) delivers high-energy beams to individualized tumor volumes identified by modern non-invasive imaging techniques (CT-scan, MRI , PET). RT elicits direct antitumoral, anti-angiogenic and immunogenic effects.Therefore, potent activation of the immune system could be confined to the irradiated tumor volume. However, these positive effects of RT are, in part, counterbalanced by immune evasion and tissue remodeling processes, limiting exploitation of its full therapeutic potential. Activation of immune checkpoints (e.g., PD-L1) and expression of TGFβ are among the most relevant undesired RT effects detected at the tumor microenvironment (TME) interface. We hypothesized that reprogramming the TME via trapping of TGFβ combined with anti-PD-L1 immune checkpoint blockade may synergize with radiotherapy towards development of an effective in situ tumor vaccination strategy. In fact, we could show that immune activation by RT and TME reprogramming via simultaneous targeting of PD-L1/TGFβ (Bintrafuspalpha, BA) are together required for induction of a CD8+ T cell-dependent tumor-specific immunity. In line, BART combination not only improved tumor responses at the irradiated tumor sites, but also led to regression of abscopal metastases, outside the irradiation field.
What was the most surprising finding of this study for you?
Perhaps the most surprising finding was that BA, but not TGFβ trap alone was able to attenuate undesired late radiation-induced normal tissue toxicity (lung fibrosis). Single-cell analysis revealed that key TGFβ-expressing subpopulations (i.e. clusters of endothelial cells and M2-like lipofibroblasts) also expressed PD-L1. Therefore, in addition to combining two IO modalities, the anti-PD-L1 arm of BA may target TGFβ trap to the relevant populations. This discovery may now open the field for development of next generation IO drugs with enhanced efficacy and possibly reduced toxicity by selective targeting of therapeutic modules to specific cellular compartments, rather than global inhibition or activation of signaling cascades.
What was the coolest thing you’ve learned (about) recently outside of work?
Among a plethora of lessons we all learned from the COVID-19 pandemic, time-lapse tracking of SARS-COV2 evolution was/is intriguing and instructive. It started with the appearance of the D614G mutation in Europe that would later dominate the global pandemic. It continued with the formation of the N501Y variant in UK/alpha and almost simultaneously the beta/gamma variants in South Africa and Brazil with additional mutations in 484 and 417 positions of the RBD. The community was preparing for these converging mutations and anticipated a narrow evolutionary landscape for viral escape. Within only a couple of months, the world is now dominated by the delta variant 617.2 that started its rise in India and lacks all these mutations. The delta variant, rather, combines the two mutations previously found in California (L452R) and Mexico (T478K). Time will show if the predicted convergence of immune-escape mutations will resurrect once the global immunization rate increases, or novel residues or completely new variants will emerge. The challenge in prediction of the evolutionary landscape of a relatively genetically stable coronavirus system teaches us that we need to substantially gear up our knowledge to understand or even predict the evolution of the tremendously more complex and genetically unstable tumor model system.