The epithelium of the thymus plays a crucial role in T cell maturation, providing positive selection for MHC recognition by cortical thymic epithelial cells and negative selection for self-antigens by medullary thymic epithelial cells (mTECs) and antigen-presenting cells. mTECs ectopically express peripheral-tissue antigens (PTAs), and the transcription factor Aire has been implicated as a driver of PTA expression. In research recently published in Cell, Michelson et al. performed single-cell analyses for transposase-accessible chromatin sequencing (scATACseq) to evaluate the mechanisms of PTA expression in mTECs.
To research how chromatin accessibility affects PTA expression in individual mTECs, the researchers performed scATAC-seq on purified MHC-II-high mTECs (mTEChi) from Aire+/+ and Aire-/- mice. The obtained data were segregated in 13 clusters, which were annotated with known mTEC subtypes based on chromatin accessibility at marker genes. Most cells (clusters 4-7) had strong accessibility at the Aire gene body and loci associated with antigen presentation. These cells were appointed as Aire-stage mTECs, and these clusters were labeled as Aire-expressing or Aire-deficient based on the mice genotype in which these were found. Cluster 3 had enhanced accessibility at podoplanin (Pdpn) and Krt5, which corresponds with an immature mTEC subtype. Two clusters were between the immature and Aire-stage subtypes, suggestive of transit-amplifying TEC and their immediate progeny. The final six clusters (clusters 8-13) were identified as post-Aire mTECs, with accessibility at post-Aire markers.
Michelson et al. then researched the effect of Aire on chromatin accessibility in mTECs. When the scATAT-seq data were separated by genotype, major differences were found in cell distribution (UMAP). In the Aire+/+ mice, all Aire-stage mTECs were localized to one Aire-expressing cluster (cluster 4), while for Aire-/- mice, Aire-stage mTEC were in three Aire-deficient clusters (Clusters 5-7). The immature, transit-amplifying, and post-Aire mTECs were not affected by the presence or absence of Aire. These data suggest that Aire had a strong effect on chromatin accessibility, which was primarily seen in Aire-expressing mTECs. Quantifying chromatin accessibility changes at important genomic features in cluster 5 showed that the most substantial changes were detected at Aire binding sites and Aire-induced genes, suggesting Aire promotes chromatin accessibility at its binding sites and target genes.
Transcription factor (TF)-motif-enrichment analysis of the scATACseq data showed enrichments for specific TF families that contain lineage-defining TFs from extra-thymic tissues, such as liver and gut epithelium, skin development, and neuroendocrine and secretory cell types. In addition, immunofluorescence of thymus tissue confirmed the expression of these TF family members in thymic medullary epithelial cells.
Further assessing the importance of these lineage-defining TFs of peripheral cell types in mTECs, the researchers found that post-Aire clusters also had an accessible-chromatin landscape corresponding to the peripheral cell type of the specific TF. Clusters were therefore named after the corresponding peripheral counterpart (including keratinocyte, ciliated, and secretory/neuroendocrine mTECs), and together these peripheral-cell-mimicking mTECs were called mimetic cells.
To identify markers to enrich for mimetic cells in the mTEClo compartment, previously published Aire-lineage-tracing RNAseq data were analyzed. This revealed that the mTEClo population could be split into a pre-Aire (Pdpn+CD104+) and a post-Aire (Pdpn-CD104-) compartment. To assess whether mimetic cells in the scATACseq data had matching transcriptional profiles in the mTEClo compartment, scRNAseq was conducted on Pdpn-CD104- cells. There was a large diversity of mTECs within the post-Aire mTEClo compartment, and all mimetic cell subsets detected earlier were found, as well as additional subtypes. Overlaying published gene signatures from extra-thymic cell types revealed clear compartmentalization of mimetic cell subsets, with the most differential genes for each subtype corresponding with key marker genes and lineage-defining TFs for peripheral cell types. Re-analysis of RNAseq data of human fetal thymic epithelium showed expression of mimetic cell gene signature orthologues in subtypes of mimetic cells, suggesting these exist not only in mice, but also in humans.
Michelson et al. then investigated the role of lineage-defining TFs in mimetic cells. The chromatin binding of several lineage-defining TFs was mapped and compared to published Aire binding data. The binding of lineage-defining TFs was restricted to pan-mTEC open chromatin regions (OCRs) and their respective mimetic-cell OCRs, while Aire could bind all OCRs. Aire could enhance the binding of some of the lineage-defining TFs, but was not required.
To assess whether lineage-defining TFs were necessary for mimetic cell accumulation, the researchers focused on microfold mTECs (analogous to mature gut M cells). scRNAseq showed that these cells expressed high levels of transcripts encoding classic gut M cell markers. Immunofluorescence of thymic tissue showed that GP2+ cells (a marker used to identify gut M cells) were confined to medullary islets, had dendritic morphology, and encircled lymphocytes, similar to gut M cells. Purified gut M cells were found to share similar upregulation of the microfold signature. Therefore, microfold mTECs upregulated transcriptional signatures resembling gut M cells, but kept their mTEC identity.
SpiB and Sox8 are defining TFs of peripheral M cells, and RNAseq data showed microfold mTEC expressed high levels of the genes encoding these TFs, and SpiB- and Sox8-deficient mice had a significant loss of microfold mTECs. To assess whether the requirement for these TFs was TEC-intrinsic, thymic grafting experiments were conducted in which thymi from SpiB- and Sox8-deficient newborn mice were transplanted into WT mice. While control mice developed microfold mTECs, the deficient thymi had major defects in microfold mTEC accumulation. In the deficient mice, the Pdpn-CD104- mTEClo cells showed loss of microfold-associated transcripts. Therefore, SpiB and Sox8 were necessary for microfold mTEC accumulation and associated PTA expression in thymic epithelium.
Clustering scRNAseq data from Pdpn-CD104- mTEClo from WT, SpiB-/-, Sox8-/-, and Aire-/- mice revealed clear differences in cell distribution between the genotypes. Microfold mTEC were nearly missing in the SpiB- and Sox8-deficient mice, while other mimetic cell types were unaffected. Aire deficiency resulted in an accumulation of mimetic cells, and Aire was not required to activate mimetic-cell PTAs.
Finally, the researchers assessed whether mimetic cells were essential for antigens involved in the negative selection of autoreactive T cells and positive selection of Tregs. A model antigen (YFP) was expressed in various thymic cell compartments (ciliated, muscle, or total mTECs), mice were immunized with YFP, and cognate T cells were quantified using tetramers. Expression of YFP in each of these cell types resulted in induction of T cell tolerance to YFP, with the strongest tolerance arising from the highly frequent total mTECs.
This research shines new light on the process and cells involved in the thymus that mediate T cell tolerance to self-antigens and shed new light on the function of Aire.
Write-up by Maartje Wouters, image by Lauren Hitchings.
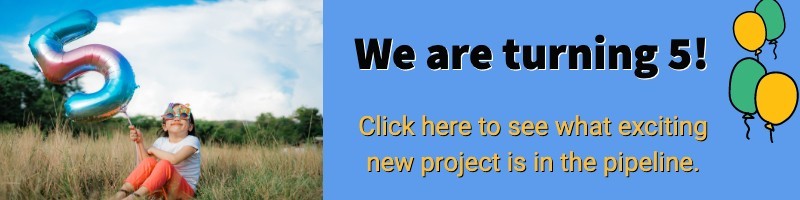
Meet the researcher
This week, first author Daniel Michelson and lead author Diane Mathis answered our questions.
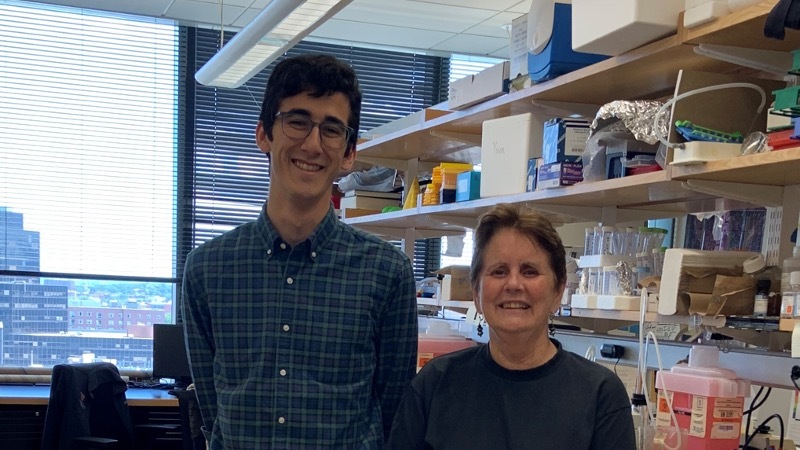
What was the most surprising finding of this study for you?
Initially, we were very surprised to discover cells in the thymus that looked like microfold (M) cells, a rare and ultra-specialized cell type from the gut. But when we dug deeper into our data, and also took into account the fact that tuft cells, another rare mucosal cell type, had been recently described in the thymus, we realized that it wasn’t just M cells in the thymus, or just tuft cells, but rather this whole constellation of thymic epithelial cells mimicking different peripheral cell types for the purposes of T cell tolerance. That was a big “aha!” moment for us and really inspired the rest of the study of these peripheral cell mimics, which we termed "mimetic cells."
What is the outlook?
We’ve really only just begun to understand the roles of thymic mimetic cells. So from a basic science perspective, we want to develop a more comprehensive understanding of each of the different mimetic cell types: their provenances, dependencies, effects on T cells and other thymic cells, and relationships to other mechanisms of immune self-tolerance. Importantly for translation, we also want to understand what sorts of immune defects arise when different mimetic cells are absent or dysfunctional. These studies may help us better understand why certain autoimmune diseases (like inflammatory bowel disease or type 1 diabetes) arise and, in turn, help us develop new approaches to treat these diseases.
What was the coolest thing you’ve learned (about) recently outside of work?
Diane: I recently learned you can grow mushrooms in your living room (on purpose).
Daniel: I recently learned that you can tell the quality of a Parmesan cheese by X-raying it.